Unveiling the Nexus of Quantum Computing and Cryptography: Navigating the Quantum Era
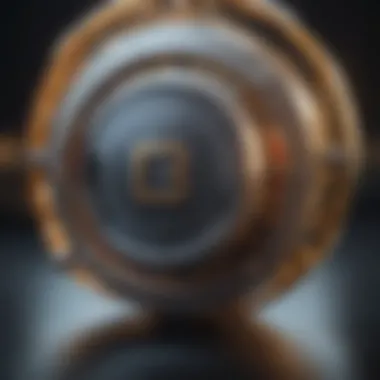
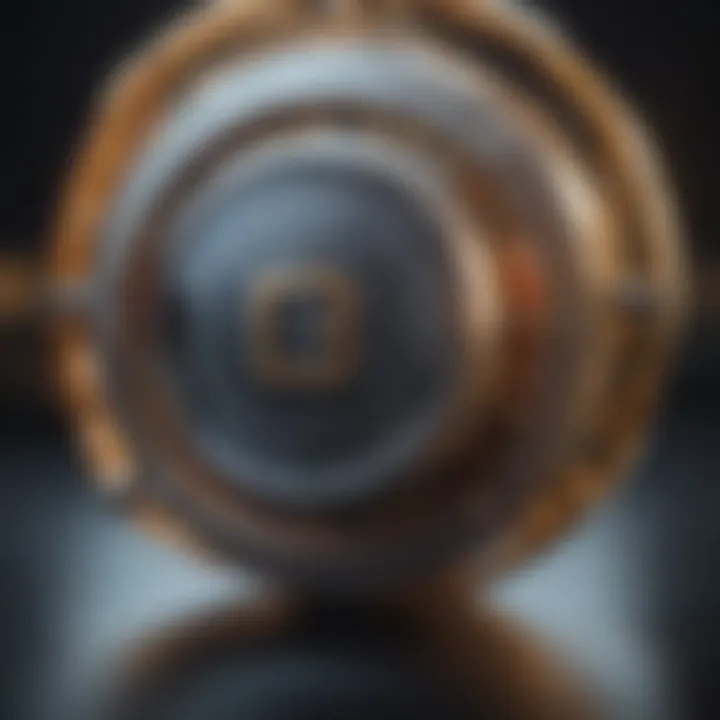
What is quantum computing and crypto:
Origins and Usage:
Quantum computing and cryptography have a fascinating origin story, with contributions from notable figures like Richard Feynman, Paul Benioff, and David Deutsch. These pioneers laid the groundwork for quantum computing by highlighting the potential of quantum mechanics for computation. In the realm of cryptography, individuals such as Whitfield Diffie and Martin Hellman revolutionized data security with the concept of public-key cryptography.
Varieties and Applications:
When it comes to quantum computing, there are various types of machines, including quantum annealers and universal quantum computers. Each serves a specific purpose, from optimizing complex optimization problems to simulating quantum phenomena that traditional computers struggle with. As for cryptography, there is a wide range of cryptographic algorithms used for data encryption, authentication, and key exchange. These algorithms play a crucial role in securing sensitive information in digital communications.
Tokenomics and Ecosystem:
The tokenomics of quantum computing and cryptography are intricate, involving a range of tokens that facilitate transactions within their respective ecosystems. Tokens such as QuantumCoin and CryptoSafe are essential for incentivizing network participants and securing blockchain networks. Additionally, quantum computing and cryptography ecosystems are supported by tools like quantum key distribution systems, cryptographic libraries, and secure communication protocols. These tools enhance the efficiency and security of quantum-based computations and data protection.
Swapping vs. Trading:
In the realm of quantum computing and cryptography, the distinction between swapping and trading is significant. While trading involves the exchange of assets based on market values, swapping refers to exchanging tokens or cryptographic keys within a decentralized network. Swapping is crucial for maintaining data integrity and network security in blockchain systems. Understanding the differences between swapping and trading is essential for engaging effectively in quantum-based transactions and ensuring the robustness of cryptographic protocols.
Acquisition Process:
To buy quantum computing and cryptographic tokens, individuals can utilize cryptocurrency exchanges or decentralized platforms that support these digital assets. It is essential to research and choose reputable exchanges that offer secure transactions and a wide selection of quantum-related tokens. By following proper security protocols and conducting due diligence on exchange platforms, investors can acquire quantum computing and cryptographic assets with confidence and transparency.
Preface
Divulging into the amalgamation of quantum computing and cryptography propels us into the realm where state-of-the-art technology interfaces with the robust bastion of secure communication. This section acts as the gateway, laying the foundation for comprehending the nuanced synergy between these two disciplines and the subsequent evolution of cybersecurity. By scrutinizing the juxtaposition of quantum computing and cryptographic frameworks, we unravel a tapestry of complexity and sophistication. The crux lies in deciphering how quantum advancements have both revolutionized and imperiled conventional cryptographic methods, sparking a pivotal shift towards quantum-safe encryption protocols. Delving into this dynamic domain unveils a landscape filled with challenges, exigencies, and opportunities, necessitating a proactive stance in fortifying digital fortresses against quantum incursions.
Understanding Quantum Computing
In the intricate realm of quantum technology, understanding quantum computing is paramount. It serves as the foundational framework upon which the rest of the discussion on quantum computing and its intersection with cryptography is built. Quantum computing, unlike classical computing, operates based on quantum bits or qubits, revolutionizing computational processes and capabilities in profound ways. As we delve into the specifics of quantum computing, various elements come to light, shedding insight into the potential and challenges this technology presents.
Fundamentals of Quantum Computing
Superposition and Entanglement
Superposition and entanglement are pivotal concepts in quantum computing, defining its uniqueness and power. The ability of qubits to exist in multiple states simultaneously through superposition allows for exponential computational possibilities. Furthermore, entanglement enables the correlation of qubits regardless of the distance between them, facilitating unprecedented coherence and information processing. These features distinguish quantum computing from its classical counterpart, opening doors to complex calculations and algorithms that were previously unimaginable.
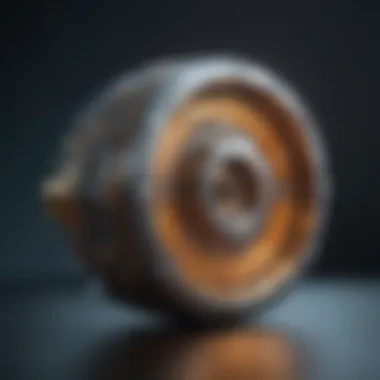
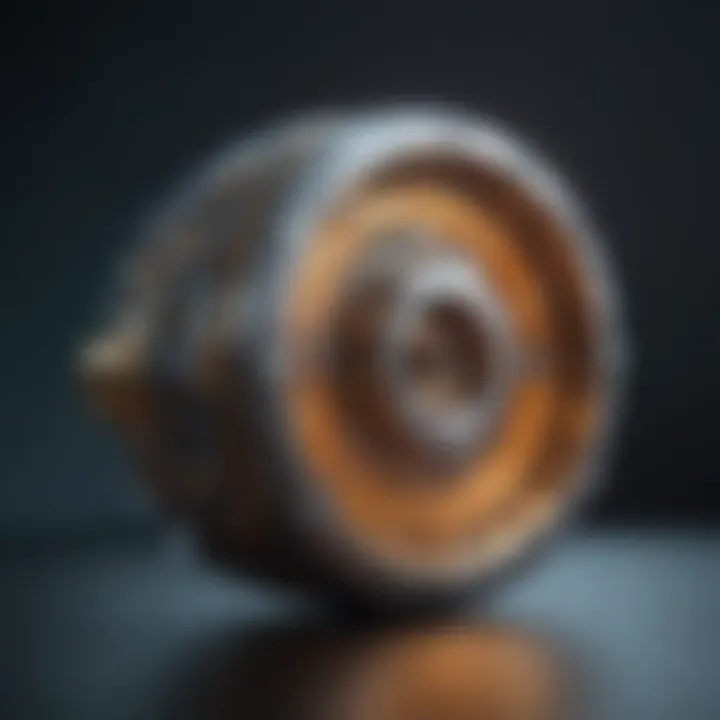
Quantum Bits (Qubits)
Quantum bits, or qubits, form the fundamental unit of quantum information, embodying both 0 and 1 simultaneously due to superposition. This duality gives qubits their quantum advantage, enabling parallel processing and intricate calculations that surpass classical binary systems. Despite their immense potential, qubits are highly sensitive to environmental interferences, posing a challenge in maintaining coherence and stability for prolonged computations. However, advancements in qubit designs and error-correction techniques aim to overcome these limitations, paving the way for practical quantum applications.
Quantum Gates
Quantum gates are akin to classical logic gates but operate on qubits, manipulating their states through quantum operations. These gates perform transformations essential for quantum algorithms, such as quantum Fourier transforms and quantum phase estimation. By harnessing quantum gates effectively, computations can be streamlined, enabling the execution of complex operations with remarkable efficiency. Nonetheless, the intricate nature of quantum gates requires precision in implementation to ensure accuracy and reliability, underscoring the delicate balance between quantum parallelism and error mitigation.
Quantum Supremacy
Achieving Quantum Advantage
Achieving quantum advantage signifies surpassing the computational capabilities of classical systems, heralding a new era in information processing. Quantum supremacy, a milestone in quantum computing, marks the moment when quantum devices outperform classical supercomputers on specific tasks. This leap showcases the transformative potential of quantum technologies in addressing complex problems exponentially faster, revolutionizing fields like optimization, cryptography, and simulation.
Applications and Implications
The applications of quantum computing span diverse domains, from cryptography to drug discovery, leveraging quantum algorithms to accelerate computations and solve intricate problems efficiently. Quantum supremacy not only propels scientific research but also raises security concerns in cryptography due to its potential to crack conventional encryption methods swiftly. However, these implications drive the urgency for quantum-safe cryptographic solutions, heralding a paradigm shift in cybersecurity strategies to combat emerging threats effectively.
Cryptography in the Digital Age
Cryptography is a crucial element in the digital landscape, serving as the cornerstone of secure communication and data protection. In the digital age, where information is the new currency, cryptography plays a pivotal role in safeguarding sensitive data from malicious entities. The utilization of cryptographic techniques ensures privacy, integrity, and authenticity in various online transactions and communications. Without robust cryptography, the digital realm would be vulnerable to cyber breaches and threats, jeopardizing both individual privacy and national security.
Classical Cryptography
Symmetric Encryption
Symmetric encryption is a fundamental aspect of classical cryptography, employing a single shared key for both encryption and decryption processes. This key symmetry streamlines communication between parties, offering a simplistic and efficient method for securing data. The symmetrical nature of this encryption technique enhances processing speeds and reduces computational overhead, making it ideal for scenarios requiring rapid data encryption and decryption. However, the primary limitation of symmetric encryption lies in key distribution, as sharing the encryption key securely between communicating parties poses a significant challenge.
Asymmetric Encryption
Asymmetric encryption, in contrast to symmetric encryption, utilizes a pair of keys (public and private) for encryption and decryption tasks. This dual-key approach adds an extra layer of security to the communication process, as the public key can be freely shared while the private key remains confidential. Asymmetric encryption eliminates the key distribution issue present in symmetric encryption, enhancing security in digital transactions. Despite its security advantages, asymmetric encryption generally requires more computational resources compared to symmetric encryption, potentially impacting processing speeds in resource-constrained environments.
Hash Functions
Hash functions form an integral part of cryptographic systems, generating fixed-length outputs from input data of variable lengths. These functions play a critical role in ensuring data integrity and authentication, as any modification to the input data results in a distinct hash value. Hash functions are widely used in digital signatures, password storage, and data verification processes due to their deterministic output and collision resistance properties. However, the prevalence of preimage attacks poses a notable challenge to the security of hash functions, emphasizing the need for continuous algorithmic advancements to mitigate cryptographic vulnerabilities.
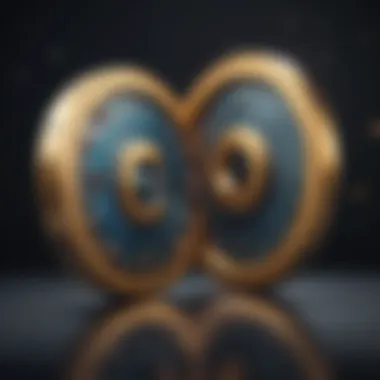
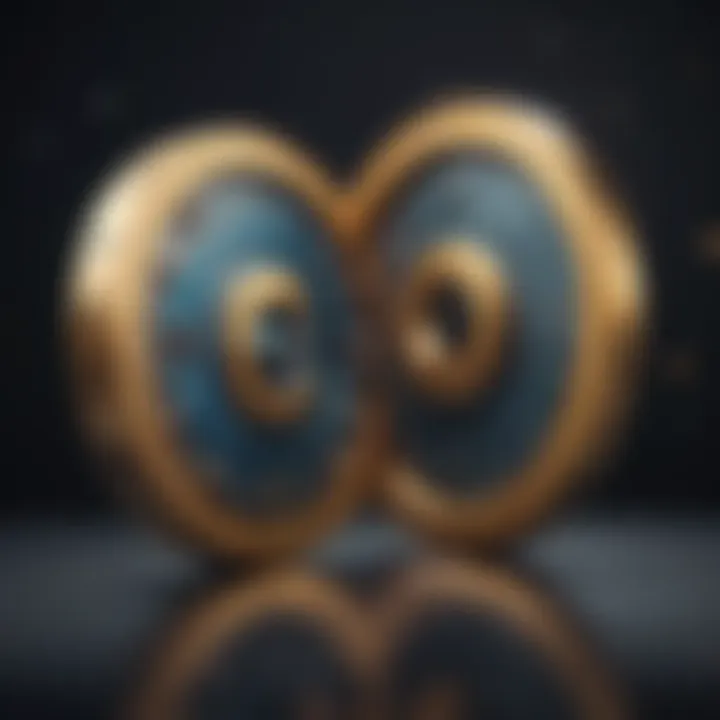
Challenges of Classical Cryptography
Vulnerabilities to Quantum Attacks
Classical cryptographic systems, including symmetric and asymmetric encryption techniques, face increasing vulnerabilities in the advent of quantum computing. Quantum algorithms such as Shor's Algorithm possess the capability to efficiently factor large numbers, compromising the security of widely-used encryption schemes like RSA. The development of quantum-resistant encryption algorithms becomes imperative to counter the impending threat of quantum attacks on classical cryptographic systems.
Limitations in Post-Quantum Security
Post-quantum security refers to the resilience of cryptographic algorithms against quantum-enabled attacks. The limitations in achieving robust post-quantum security stem from the complexity of designing encryption schemes that can withstand quantum threats while maintaining compatibility with existing systems. Balancing security, efficiency, and interoperability remains a significant challenge in the advancement of post-quantum cryptography, necessitating extensive research and collaboration within the cryptographic community to address these critical limitations.
Impact of Quantum Computing on Cryptography
In this pivotal segment delving deeply into the convergence of quantum computing and cryptography, we unravel the substantial ramifications of the quantum realm on traditional cryptographic methodologies. The robust influence of quantum computing on cryptography cannot be understated; it marks a paradigm shift in how we approach secure communication and data protection. As quantum technologies advance, the encryption landscape undergoes a metamorphosis, urging the adaptation of quantum-safe encryption solutions to thwart potential breaches.
Shor's Algorithm and RSA Encryption
Breaking RSA Encryption
Delving into the intricate realm of Breaking RSA Encryption illuminates a critical juncture in cryptographic evolution. This facet of encryption disruption brought forth by Shor's Algorithm poses a formidable challenge to the conventional security protocols the digital realm relies on. The essence of Breaking RSA Encryption lies in its ability to efficiently factorize large semiprime numbers, exposing the vulnerability of RSA encryption to quantum threats. Its significance in this discourse lies in reshaping the cybersecurity landscape, pushing for the development of robust quantum-resistant cryptographic frameworks.
Implications for Secure Communication
Assessing the Implications for Secure Communication encapsulates the crux of quantum's impact on data privacy and integrity. The pivotal implication stems from the compromised security afforded by traditional encryption schemes in the wake of quantum prowess. As quantum algorithms break down conventional cryptographic barriers, the necessity for quantum-safe communication protocols intensifies. Secure communication mandates a quantum-ready infrastructure to thwart potential intercepts and breaches, underscoring the urgency for innovative encryption strategies designed to counter quantum incursions.
Grover's Algorithm and Cryptanalysis
Speeding Up Brute Force Attacks
Delving into the realm of Speeding Up Brute Force Attacks sheds light on the efficiency revolutionized by Grover's Algorithm. The essence of speeding brute force attacks through quantum acceleration underscores a fundamental shift in cryptanalysis methodologies. This disruptive capability bolsters the computing power wielded by malicious actors, necessitating counteractive measures to fortify encryption defenses. While the accelerated brute force tactics pose a threat, they also propel cryptographic innovation towards resilient quantum-safe encryption standards.
Mitigating Strategies
Exploring Mitigating Strategies ushers in a proactive approach towards nullifying the adversarial potential unleashed by quantum cryptanalysis. The essence of mitigating strategies lies in the development of adaptive cryptographic frameworks designed to thwart quantum-based decryption attempts. By integrating dynamic encryption protocols and quantum-aware security mechanisms, organizations can fortify their data assets against potential quantum breaches. The efficacy of these strategies lies in their ability to anticipate and counteract evolving cyber threats, ensuring data confidentiality and integrity remain paramount in the quantum era.
Quantum-Safe Cryptography
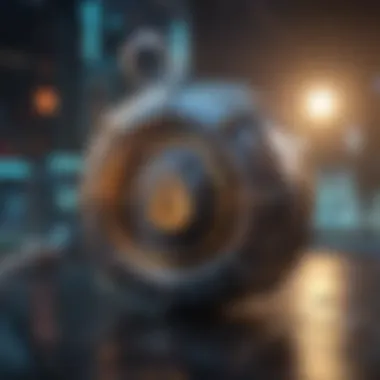
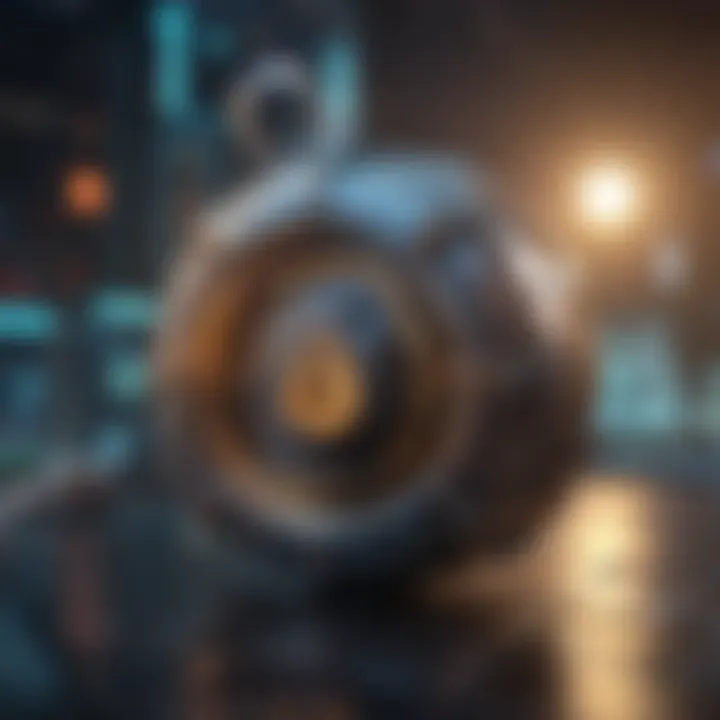
Quantum-Safe Cryptography stands at the forefront of cryptographic research and development, poised to tackle the encryption challenges posed by quantum computing advancements. In this intricate landscape of quantum and cryptography, the significance of Quantum-Safe Cryptography lies in its ability to provide secure communication channels resistant to quantum attacks. By employing cryptographic algorithms that can withstand the computing power of quantum systems, Quantum-Safe Cryptography ensures data confidentiality and integrity in a post-quantum era. One of the main benefits of Quantum-Safe Cryptography is its proactive approach towards preemptively addressing the vulnerabilities of current cryptographic methods to quantum threats. Moreover, the considerations surrounding Quantum-Safe Cryptography extend to the necessity of future-proofing data security measures against exponential leaps in computing capabilities.
Lattice-Based Cryptography
Security Post-Quantum
Security Post-Quantum in lattice-based cryptography represents a pivotal advancement in ensuring cryptographic resilience beyond the scope of quantum computing. The unique characteristic of Security Post-Quantum lies in its reliance on mathematical challenges that cannot be efficiently solved by quantum algorithms, thereby fortifying the encryption process against quantum decryption attempts. In the context of this article, Security Post-Quantum emerges as a strategic choice due to its intrinsic ability to protect sensitive information effectively in a quantum-threat landscape. The advantage of Security Post-Quantum cryptography lies in its ability to offer long-term cryptographic security, safeguarding data transmissions well into the future. However, it also presents challenges in terms of increased computational requirements and complexities in implementation.
Advantages and Challenges
The exploration of Advantages and Challenges in lattice-based cryptography unveils a dual-faced landscape of cryptographic possibilities. The key characteristic of Advantages and Challenges revolves around the adaptability of lattice-based cryptography to quantum-resistant encryption paradigms. This versatility makes it a popular choice for enhancing data security in the context of this article on Quantum-Safe Cryptography. The unique feature of Advantages and Challenges lies in its capacity to foster innovation in cryptographic protocols while simultaneously addressing the potential drawbacks of quantum computing. However, this duality also introduces challenges related to key distribution and algorithmic efficiency, requiring meticulous considerations for practical implementation
Code-Based Cryptography
Error-Correcting Codes
Error-Correcting Codes play a pivotal role in fortifying cryptographic systems against data corruption or tampering, contributing significantly to the overall robustness of encryption mechanisms. The key characteristic of Error-Correcting Codes lies in their ability to detect and rectify errors in transmitted data, ensuring the accuracy and integrity of cryptographic operations. This feature makes Error-Correcting Codes a valuable choice for this article on Quantum-Safe Cryptography due to its role in maintaining data authenticity amidst potential quantum interference. The advantages of Error-Correcting Codes lie in their capacity to enhance data reliability and fault tolerance, mitigating risks associated with quantum computational errors. However, challenges may arise in optimizing error-correction algorithms for quantum-resistant applications
Resistance to Quantum Attacks
Resistance to Quantum Attacks serves as a critical pillar in the realm of quantum-safe cryptography, focusing on developing encryption techniques impervious to quantum decryption methods. The key characteristic of Resistance to Quantum Attacks lies in its ability to counter quantum computational threats through robust cryptographic frameworks designed to withstand quantum algorithms. This characteristic makes Resistance to Quantum Attacks a preferable choice for enhancing data security in the context of this article on Quantum-Safe Cryptography. The unique feature of Resistance to Quantum Attacks resides in its capability to neutralize the efficacy of quantum computing in compromising data confidentiality and integrity. However, challenges associated with implementing resistance mechanisms against evolving quantum strategies necessitate continuous advancement and adaptation to ensure cryptographic resilience in the quantum computing paradigm
Future Directions and Challenges
Quantum Key Distribution
Unforgeable Communication
When delving into the specifics of quantum key distribution, the concept of unforgeable communication surfaces as a cornerstone of secure data transmission. Unforgeable communication stands out for its unparalleled ability to ensure that data exchanged between parties remains intact and unaltered. This characteristic distinguishes it from conventional encryption methods, offering a level of security that is inherently robust against tampering or interception attempts. In the context of this article, unforgeable communication emerges as a foundational element in forging quantum-safe encryption protocols. Its unique feature lies in the utilization of quantum principles to create cryptographic keys that are impervious to eavesdropping or malicious manipulation. While unforgeable communication presents a promising solution for enhancing data security, it also brings to light the complexities involved in integrating quantum concepts into practical encryption frameworks.
Quantum-Secure Protocols
Shifting focus to quantum-secure protocols, their significance in the overarching theme of quantum computing and cryptography comes to the fore with striking clarity. These protocols play a pivotal role in establishing secure channels for data exchange in the quantum realm, safeguarding sensitive information from unauthorized access or decoding. The key characteristic of quantum-secure protocols lies in their capability to leverage quantum properties such as superposition and entanglement to fortify communication channels against external threats. Their unique feature encompasses the sophisticated algorithms and mechanisms employed to authenticate users and ensure the integrity of transmitted data. While quantum-secure protocols offer a beacon of hope for secure digital communication, their implementation poses challenges related to compatibility and scalability within existing network infrastructures.
Standardization and Adoption
Transition to Quantum-Resistant Standards
Within the narrative of standardization and adoption, the transition to quantum-resistant standards emerges as a critical juncture in the evolution of cybersecurity practices. This aspect plays a pivotal role in establishing a uniform set of protocols and encryption algorithms that are resilient against quantum-based attacks. The key characteristic of transitioning to quantum-resistant standards revolves around the creation and adoption of cryptographic methods that can withstand the computational power of quantum adversaries. This transition is not merely a choice but a necessity in safeguarding sensitive data and communications in an era dominated by quantum advancements. The unique feature of quantum-resistant standards lies in their ability to future-proof encryption mechanisms, ensuring long-term security in the face of exponential quantum computing capabilities. While the advantages of transitioning to such standards are evident, the challenges of compatibility and widespread adoption pose significant hurdles that must be addressed for seamless integration into global cybersecurity frameworks.
Global Initiatives
In exploring global initiatives within the realm of quantum computing and cryptography, a panorama of collaborative endeavors and strategic endeavors materializes. These initiatives underscore the collective effort of governments, industries, and research institutions in fortifying cybersecurity measures in the quantum age. The key characteristic of global initiatives lies in their ability to transcend geographical boundaries and foster a unified approach towards developing quantum-safe encryption standards. The unique feature of such initiatives embodies the spirit of cooperation and knowledge-sharing, essential components in tackling the multifaceted challenges posed by quantum computing advancements. While global initiatives hold the promise of standardized security protocols and interoperable encryption frameworks, the road to universal acceptance and implementation is paved with obstacles related to geopolitical interests, technological disparities, and regulatory frameworks.